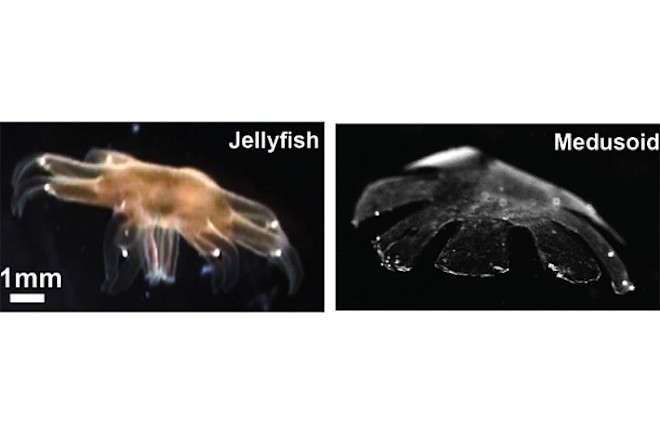
Kit Parker has built an artificial jellyfish out of silicone and muscle cells from a rat heart. When it’s immersed in an electric field, it pulses and swims exactly like a real jellyfish. The unusual creature is part of Parker’s efforts to understand the ways in which muscles work, so that he can better engineer heart tissue. And it has a bizarre intended purpose: Parker wants to use it to test heart drugs. I wrote about his work for Nature, so head over there for the main story. Meanwhile, here’s my full interview with Parker about the jellyfish. He’s a fantastic interviewee – you’ve got to imagine him almost shouting this stuff. Building a jellyfish using rat tissue isn’t exactly a typical everyday idea. Where did it come from? My group does cardiovascular research and I spend a lot of time thinking about building tools for early-stage drug discovery. We’re known for making actuators and things you can measure contractility with, and using micro-scale tissue engineering to build tissues on chips. Several years ago, I got really frustrated with the field. Drug companies are screaming because their drug pipelines are running dry. We don’t have good ways of treating a lot of these heart diseases in the clinic. It dawned on me that probably the reason why is that we’re failing to understand the fundamental laws of muscular pumps. I started looking around for inspiration in a simpler system. This was late 2007, and I was visiting the New England aquarium. I saw the jellyfish display and it hit me like a thunderbolt I thought: I know I can build that. That spring, we had a visitor: John Dabiri from CalTech, a famous fluid mechanician. He does a variety of propulsion studies on various species. He was walking down the hall and I grabbed him and said: John, I think I can build a jellyfish. He didn’t know who I was. He looked at me like I had a horn growing out of my head but I was pretty excited and waving my arms, and I think he was afraid to say no. So, he said yeah. He sent a graduate student Janna Nawroth to my lab for four years. Three of my postdocs who are on that paper are now professors – this is the best of the best that we put on that project. And what did you actually do? We took a jellyfish, and did a bunch of studies to understand how it activates its muscles. We studied its propulsion and we made a map of where every single cell was. We used a software programme that we had developed a few years ago, borrowed from law enforcement agencies for doing quantitative analysis of fingerprints, and we used it to analyse the protein networks inside the cells. We found something very interesting right away: the electrical signals that the jellyfish uses to coordinate its pumping are exactly like that of the heart. In the heart, the action potential [electrical signal that travels along nerves – Ed] propagates as a wave through cardiac muscle. That’s how you get this nice, smooth contraction. The activation has to spread like when you drop a pebble in water. The same thing happens in the jellyfish, and I don’t think that’s by accident. My bet is that to get a muscular pump, the electrical activity has got to spread as a wavefront After we had the map of where every cell was, we took a rat apart and rebuilt it as a jellyfish. Why study jellyfish? The one that we used is a juvenile – it’s like a thin monolayer of cells. It’s a very simple structure to build. The great thing about this is that most tissue engineering is just arts and crafts. We throw cells together and we say, ‘It looks like a liver; there’s a bunch of cells’. Or we throw heart cells together and hope that we build bits of heart. But if I’m building an aircraft or bridge, we don’t just throw concrete and aluminium and alloys together. We do mechanical testing on the substrates. We have mathematical models and computer simulations to understand the flight of the aircraft. We know how the bridge is going to work. Some engineers build out of copper or concrete or steel. I build things out of cells. If I’m going to be an engineer rather than an artist, I’m going to need to build quality control methods into what I’m doing. Nobody is going to get into an airplane unless they’ve done computer simulations and assumed that they’ve manufactured this within allowable tolerance. It’s not just guesswork. No one’s going to want a tissue-engineered heart or other organ put into their body unless they’ve got some manufacturers’ specification. The great thing about the jellyfish is that you can do all these highly quantitative propulsion studies. That’s why I had to have John Dabiri’s team with this – they’re the best in the world at biological propulsion. And we were able to match quantitatively match the exact same propulsion characteristics in our medusoid – our engineered jellyfish – as the real one. The most interesting thing is that the mouth of the jellyfish is inside the bell. In order to feed itself, it creates a vortex on the power stroke that throws particulate matter up towards its mouth. We thought if we’re good, if we’re really good at this, we’re going to recreate that vortex, and we did. We found that it depended on some very precise organisation of the protein networks inside the cells. The whole idea was to bring engineering design methodology with tissue engineering, with a very rigorous set of parameters to show that our tissue-engineered jellyfish is very much a jellyfish. Morphologically, we’ve built a jellyfish. Functionally, we’ve built a jellyfish. Genetically, this thing is a rat. So, the jellyfish isn’t the endpoint. The point of building it, and getting it to behave exactly like a normal jellyfish, is to show how much you understand about how the cells work. Is that it? That’s right, but it depends on the lens through which you do this. For the marine biologist who’s interested in how jellyfish swim, we’ve demonstrated how important the muscular structure is and the protein alignment inside these cells for the jellyfish to survive and feed itself. The jellyfish scientist looks at this different rather than someone who’s trying to mimic biological propulsion. They look at this as how do you build something that can propel itself with this peristaltic pumping. The tissue engineer looks at this as applying the tissue engineering methodology to the highest possible standard to tissue engineering, which hadn’t been done before. If you’re a cardiovascular physiologist or a company doing discovery, you look at this and say: wow, for years, all we’ve measure in a dish is contractility. But there’s a big difference between that and pumping. Now we’ve shown that we can build a muscular pump in a dish. You’ve got a heart drug? You let me put it on my jellyfish, and I’ll tell you if it can improve the pumping. The first two or three years of any drug’s lifetime is always spent in a dish. We filed a patent on this to use this and variations on it as a drug discovery assay. The next stage is to see if we can build this out of human cells. And we’ll probably build a variation on the jellyfish for actual drug-testing. In your paper, you describe the jellyfish as a synthetic organism. Usually when we talk about synthetic life forms, somebody will take an existing living cell and put new genes into the cell so that it behaves in a different manner. That’s synthetic biology but I think it’s overstating what you did. We built an animal. I think we’re taking synthetic biology to a new level. It’s not just about genes. It’s about morphology and function. So has this study got you further towards understanding the “fundamental laws of muscular pumps”? Yeah it has. The heart and your guts both have action potential wavefronts that propagate through the tissue. We’re going to try this in an octopus and squid, but my bet is that to get a muscular pump, you have to organise the electrical activity in the same way. You have this clean wavefront, not a single pulse down a one-dimensional nerve fibre. It’s got to spread as a wavefront. We also found that the muscle cells in a jellyfish are shaped freakishly differently to a cardiac muscle cell. But if you strip away the outer part of the cells, the protein networks within that cell are universally built in the same way and aligned among cellular aggregates in the same way. We think structure begets function. What I’m really pleased about is that everything that my group has learned about the heart in terms of structure and function equally applies to the jellyfish. I feel like we’re learning some fundamental biology here. Some people do basic biology by deconstructing stuff. Engineers do basic science in a different way. What we’ve done is learned something about the basic science by building it de novo. What’s next? Bait. Tissue-engineered bait. I want to go fishing and have a much better form of bait. That’s the only thing that’s going to impress my family. They could care less about this high-order science. They want to know if they can win a bass tournament. Seriously, there are lots of different things. We’re going to develop this into assays for drug discovery. That’s pretty important to use. We’re working on that. We’re looking to reverse-engineer other marine life-forms too; we’ve got a whole tank of stuff in there, and an octopus on order. We’re trying to build larger and smaller versions of the jellyfish so we can look at drug effects.